A reposting of an original article in The Scorpion and the Frog.
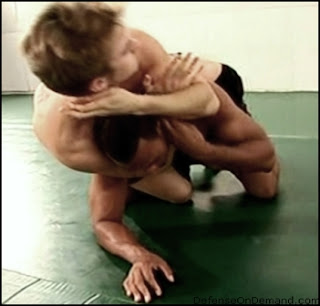 |
Photo by The Grappling Source Inc.
at Wikimedia Commons |
Being subordinated is stressful. The process of one individual lowering the social rank of another often involves physical aggression, aggressive displays, and exclusion. In addition to the obvious possible costs of being subordinated (like getting beat up), subordinated individuals often undergo physiological changes to their hormonal systems and brains. Sounds pretty scary, doesn’t it? But what if some of those changes are beneficial in some ways?
Dominance hierarchies are a fact of life across the animal kingdom. In a social group, everyone can’t be dominant (otherwise, life would always be like an episode of Celebrity Apprentice, and what could possibly be more stressful than that?). Living in a social group is more peaceful and nutritive when a clear dominance hierarchy is established.
Establishing that hierarchy often involves a relatively short aggressive phase of jostling for position, followed by a longer more stable phase once everyone knows where they fall in the social group. Established dominance hierarchies are not always stable (they can change over time or from moment to moment) and they are not always linear (for example, Ben can be dominant over Chris, who is dominant over David, who is dominant over Ben). But they do generally help reduce conflict and the risk of physical injury overall.
Nonetheless, it can be stressful to be on the subordinate end of a dominance hierarchy and these social interactions are known to cause physiological changes. Researchers Christina Sørensen and Göran Nilsson from the University of Oslo, Cliff Summers from the University of South Dakota and Øyvind Øverli from the Norwegian University of Life Sciences investigated some of these physiological differences among isolated, dominant, and subordinate rainbow trout.
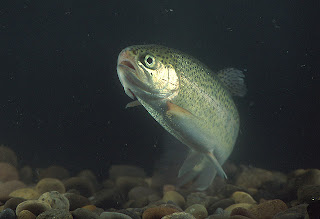 |
A photo of a rainbow trout by Ken Hammond at the USDA.
Photo at Wikimedia Commons. |
Like other salmonid fish, rainbow trout are aggressive, territorial and develop social hierarchies as juveniles. Dominant trout tend to initiate most of the aggressive acts, hog food resources, grow larger, and reproduce the most, whereas subordinate trout display less aggression, feeding, growth, and reproduction. The researchers recorded the behavior, feeding and growth rates in three groups of fish: trout housed alone, trout housed with a more subordinate trout, and trout housed with a more dominant trout. The researchers also measured cortisol (a hormone involved in stress responses), serotonin (a neurotransmitter involved in mood, the perception of food availability, and the perception of social rank, among other things) and the development of new neurons (called neurogenesis) in these same fish.
This video of two juvenile rainbow trout was taken by Dr. Erik Höglund. Here is Christina Sørensen’s description of the video: “What you see in the film is two juvenile rainbow trout who have been housed on each side of a dividing wall in a small aquarium. The dividing wall has been removed (for the first time) immediately before filming. You will see that the fish initially show interest for each other, followed by a typical display behaviour, where they circle each other. Finally one of the fish will initiate aggression by biting the other. First the aggression is bidirectional, as they fight for dominance, but after a while, one of the fish withdraws from further aggression and shows only submissive behaviour (escaping from the dominant and in the long run trying to hide... and as is described in the paper, depressed feed intake). The video has been cut to show in quick succession these four stages of development of the dominance hierarchy”.
The researchers found that as expected, the dominant trout were aggressive when a pair was first placed together, but the aggression subsided after about 3 days. Also as expected, the dominant and isolated trout were bold feeders with low cortisol levels and high growth rates, whereas the subordinate trout did not feed as well, had high cortisol levels and low growth rates. Additionally, the subordinate trout had higher serotonin activity levels and less neurogenesis than the dominant or isolated trout. These results suggest that the subordination experience causes significant changes to trout brain development (Although we can’t rule out the possibility that fish with more serotonin and less neurogenesis are predisposed to be subordinate). In either case, this sounds like bad news for subordinate brains, right? Maybe it is. Or maybe the decrease in neurogenesis just reflects the decrease in overall growth rates (smaller bodies need smaller brains). Or maybe something about the development of these subordinate brains improves the chances that these individuals will survive and reproduce in their subordination.
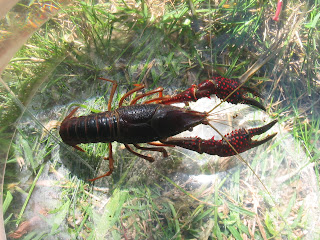 |
A crayfish raising its claws. Image by Duloup at Wikimedia. |
Research on dominance in crayfish by Fadi Issa, Joanne Drummond, and Don Edwards at Georgia State University and Daniel Cattaert at the University of Bordeaux helps shed light on this third possibility. Crayfish (which are actually not fish at all, but are freshwater crustaceans that look like small lobsters) form long-lasting and stable social hierarchies. If you poke a crayfish in the side, an isolated or dominant crayfish will turn towards whatever poked it and raise its posture and claws to confront it; A subordinate crayfish will do one of two maneuvers that involves lowering the posture and backing away from whatever poked it. Furthermore, dominant and subordinate crayfish have different neuronal activity patterns in response to being poked, and part of this difference involves differences in the activity of serotonergic neurons.
It appears that the brains of dominant and subordinate individuals function differently and part of this difference involves serotonin. This may help dominant animals to continue to behave in a dominant fashion and subordinate individuals to continue to behave in a subordinate fashion, thereby preserving the peace for the whole social group.
Want to know more? Check these out:
1. Sørensen, C., Nilsson, G., Summers, C., & Øverli, �. (2012). Social stress reduces forebrain cell proliferation in rainbow trout (Oncorhynchus mykiss) Behavioural Brain Research, 227 (2), 311-318 DOI: 10.1016/j.bbr.2011.01.041
2. Issa, F., Drummond, J., Cattaert, D., & Edwards, D. (2012). Neural Circuit Reconfiguration by Social Status Journal of Neuroscience, 32 (16), 5638-5645 DOI: 10.1523/JNEUROSCI.5668-11.2012
3. Yeh, S., Fricke, R., & Edwards, D. (1996). The Effect of Social Experience on Serotonergic Modulation of the Escape Circuit of Crayfish Science, 271 (5247), 366-369 DOI: 10.1126/science.271.5247.366
4. Issa, F., & Edwards, D. (2006). Ritualized Submission and the Reduction of Aggression in an Invertebrate Current Biology, 16 (22), 2217-2221 DOI: 10.1016/j.cub.2006.08.065